Chapters
Lodish 4th edition: Chapter 21 pages 921 - 924
Moyes and Schulte: Chapter 3 and 11
Epithelia: stomach,
intestine and kidney etc.
We are going to use a few examples of different epithelial to illustrate how
ATPase pumps and transporters work together
Epithelial cells have a specialized structure with a clear separation
between the apical surface and the basal surface
Usually the apical side faces the lumen of the stomach, intestine, kidney
etc and the basal side faces the body cavity or blood
The apical side will have a completely different complement of proteins
compared to the basal side and this difference is maintained by a series of
specialized junctions found near the apical side
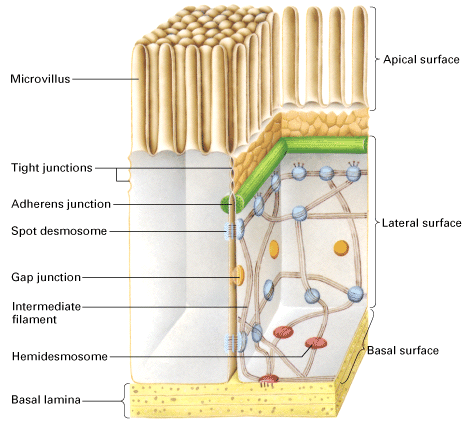
From Lodish, Molecular Cell Biology
There are two types of junctions at the apical side of vertebrate epithelial
cells. Adherens junctions that function to keep the cells in contact and
tight junctions. We will talk about tight junctions for this part.
Tight junctions are created by two sets of proteins from apposing membranes
that form a tight seal when brought into contact. A specialized set of
proteins called claudins and occludins are found at these junctions and are
necessary for its formation
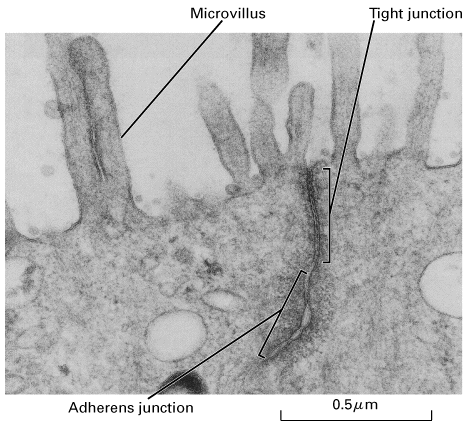
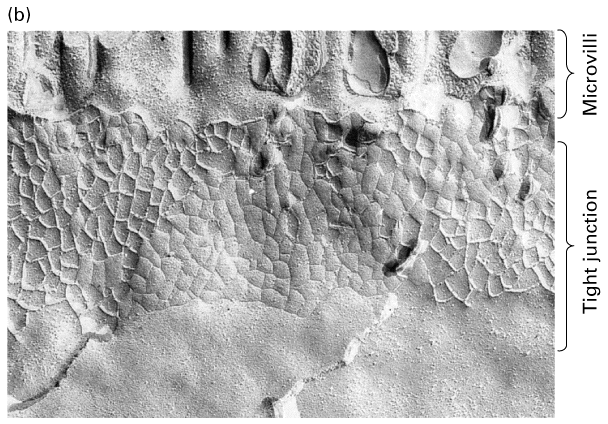
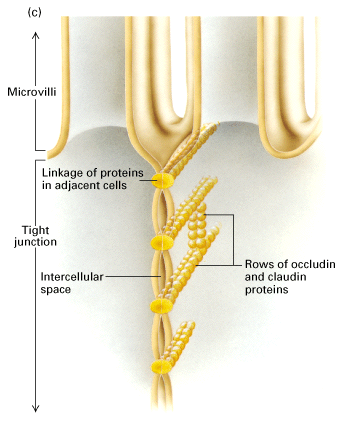
From Lodish, Molecular Cell Biology
The junction is impermeable meaning that water, ions etc can not pass
between the cells. This is an essential function of tight junctions. Just
think what would happen if your stomach lining lost its tight junctions
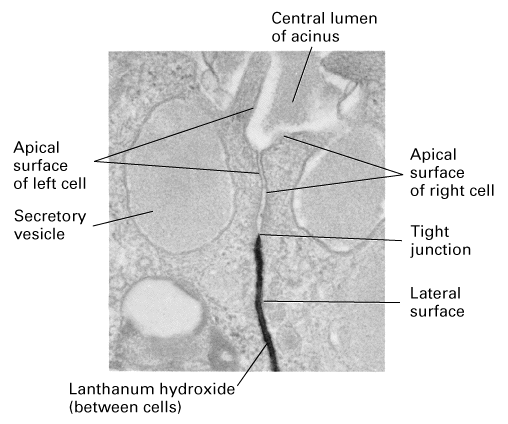
From Lodish, Molecular Cell Biology
You can test the barrier function by adding an electron dense ion like
lanthanum to one side of the epithelium and then do EM analysis. In this
example the ion can not cross the junction.
Stomach parietal
cells

From www.med.monash.edu.au
Figure 1. Schematic diagram showing the organization of the gastric mucosa,
gastric gland, gastric parietal cell and the gastric H/K ATPase.
The parietal cells are one of a group of specialized epithelia cells that line
the stomach.
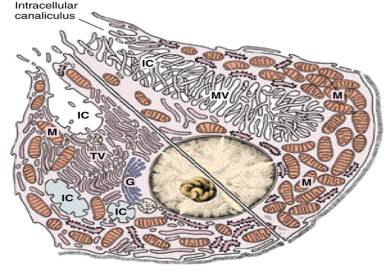
Diagram of a parietal cell. The lower half is an inactive cell the up half
an active one. Note the presence of many mitochondria (M) and the extensive
microvilli (MV) in the active cell.
From Lodish, Molecular Cell Biology
The parietal cells of the stomach generate the HCl acidic stomach fluid. The
pH of the stomach is 1 therefore the [H+] concentration is 106
times greater than inside the parietal cells themselves pH 7.
The H+/K+ ATPase is necessary to drive the H+ up a very strong concentration
gradient. Electrical potential is not altered because the export of one H+
is matched by the import of one K+. But as H+ is pumped out of the cell the
pH inside becomes alkaline. So to counteract this the HCO3-/Cl-
transporter functions to remove HCO3- (thus removing OH-) to
maintain pH. But the consequence of this is that Cl- levels rise inside the
cell so there are leak Cl- channels in the apical membrane to allow Cl-
efflux into the stomach lining and to match the loss of negative Cl- ions
the leak K+ channel allows K+ efflux through this membrane as well.
Therefore there is excess K+ ions in the stomach fluid which allows for the
H+/K+ ATPase to work in the first place. Whew!!!!
These cells are full of mitochondria to create the necessary ATP reserves
and thus these cells generate lots of CO2 which can easily be
converted to CO2 + H2O -> HCO3- + H+ to
generate the abundant H+ necessary in this cell.
Intestinal cells
and glucose transport
Movement of sugars and amino acids from the intestinal lumen into the blood
is a two-stage process.
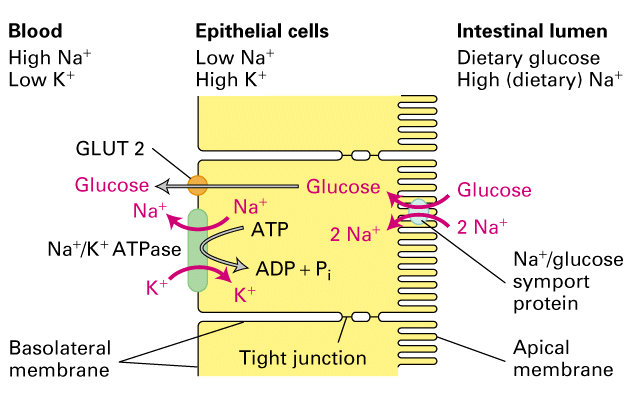
From Lodish, Molecular Cell Biology

From Alberts, Molecular biology of the cell.
Figure 11-12. Transcellular transport. The transcellular transport of
glucose across an intestinal epithelial cell depends on the nonuniform
distribution of transport proteins in the cell's plasma membrane.
The transport of glucose from the lumen of the intestine lumen to the
extracellular fluid (from where it passes into the blood) requires a number of
different transporters. Glucose is brought into the cell through the apical
domain of the membrane by a Na+ -powered glucose symporter we discussed
previously. Glucose then passes out of the cell (down its concentration
gradient) by passive transport or "facilitated diffusion" through a GLUT2
transporter in the basal and lateral membrane domains. GLUT2 is very similar
to the GLUT1 we discussed before.
NOTE: Important features to allow this include the Na+/K+ ATPase pump to
maintain the Na+ gradient (i.e. a low Na+ within the cell) and the leak K+
channel to ensure a electrical gradient.
Another important feature is the tight junctions which maintain the
permeability barrier to block leakage across the epithelium AND maintain the
polarized location of membrane proteins.
Proximal kidney
cells
The job of the kidney is to filter the blood and remove excess nitrates and
other metabolic byproducts but in addition ensure that all the important
molecules such as glucose, proteins, Na+, H2O etc are reabsorbed and
returned to the blood.
We are only going to use the reabsorption processes that occurs in the
proximal tubule of the kidney to illustrate the function of transporters in
epithelial cells. The cells that line the kidney tubules are epithelial
cells with the same polarized structure as the other epithelia discussed, ie.
distinct apical/basal domains and tight junctions.
A diagram of the kidney showing Bowman's capsule where the blood is filtered
into the kidney. The filter will not allow cells to pass but all fluid and
solutes in the blood will pass through. As the blood filtrate moves further
down the kidney tubules most solutes are reaborbed. Those that are left
behind become concentrated as the filtrate becomes urine.

From Moyes and Schulte
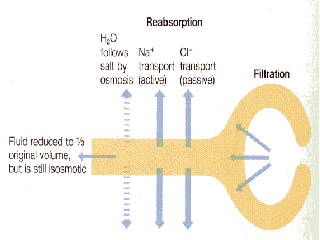
The filtrate will move along the proximal tubule and during this process
reabsorption occurs

Reabsorption is by either active or passive transport. Reabsorption consumes
a large amount of ATP and oxygen, the kidney receives ~25% of cardiac
output. The proximal tubule reabsorbs 60% of all solute, which includes 100%
of glucose and amino acids, 90% of bicarbonate and 80-90% of inorganic
phosphate, Na+ and water. All solutes are absorbed actively.
Transport of all solute is driven by the Na+/K+ ATPase on the basolateral
side of the cell which maintains a low intracellular Na+ concentration and
creates a chemical gradient for the movement of Na+ from the blood filtrate
into the cell.
Glucose
100% of glucose is reabsorbed from the blood filtrate and returned to the
blood.
This is easy in the first part of the tubule, the epithelial cells transport
glucose against a relatively small glucose concentration gradient. In this
case the cells use a 1Na+/glucose cotransporter protein. This has a high
transport rate but cannot transport glucose up a steep concentration gradient
and can only create a internal glucose concentration ¼100 times that of the
extracellular filtrate or the forming urine.
Further down the kidney tubule the epithelial cells have to remove the
remaining glucose against a more than 100-fold glucose concentration gradient.
These cells contain use the 2Na+/glucose transporter discussed above for
intestinal epithelial cells and thus can continue the transport of glucose up
a very steep concentration gradient.
H2O
H2O is absorbed passively by following solutes back into cell.
Most of the solute reabsorption is active, with water being freely permeable
and therefore moving by osmosis. When the reabsorption of solute from the
tubule occurs, there is a fall in solute concentration and hence osmotic
activity within the tubule. Water then moves because of osmotic forces to
the area outside the tubule where the concentration of solutes is higher.
Na+
The major mechanism to remove Na+ from the filtrate is to pair its movement
into the cell with the movement of other solutes. On the basal side of the
cell Na+/K+ ATPase plus the leak K+ ensures a strong concentration gradient
and negative Em for the favourable transport of Na+ into the cell from the
filtrate. The cells have used this to harness Na+ to cotransport a wide range
of solutes from the filtrate including:
- Na+/Glucose cotransporter
- Na+/amino acid cotransporter
- Na+/Cl- cotransporter
- Na+/K+ cotransporter
These are all found on the apical membrane that lines the lumen of the
proximal kidney tubule.
Osmolarity and water balance

From Molecular biology of the cell.
Figure 11-16. Response of a human red blood cell to changes in osmolarity of
the extracellular fluid. The cell swells or shrinks as water moves into or
out of the cell down its concentration gradient.
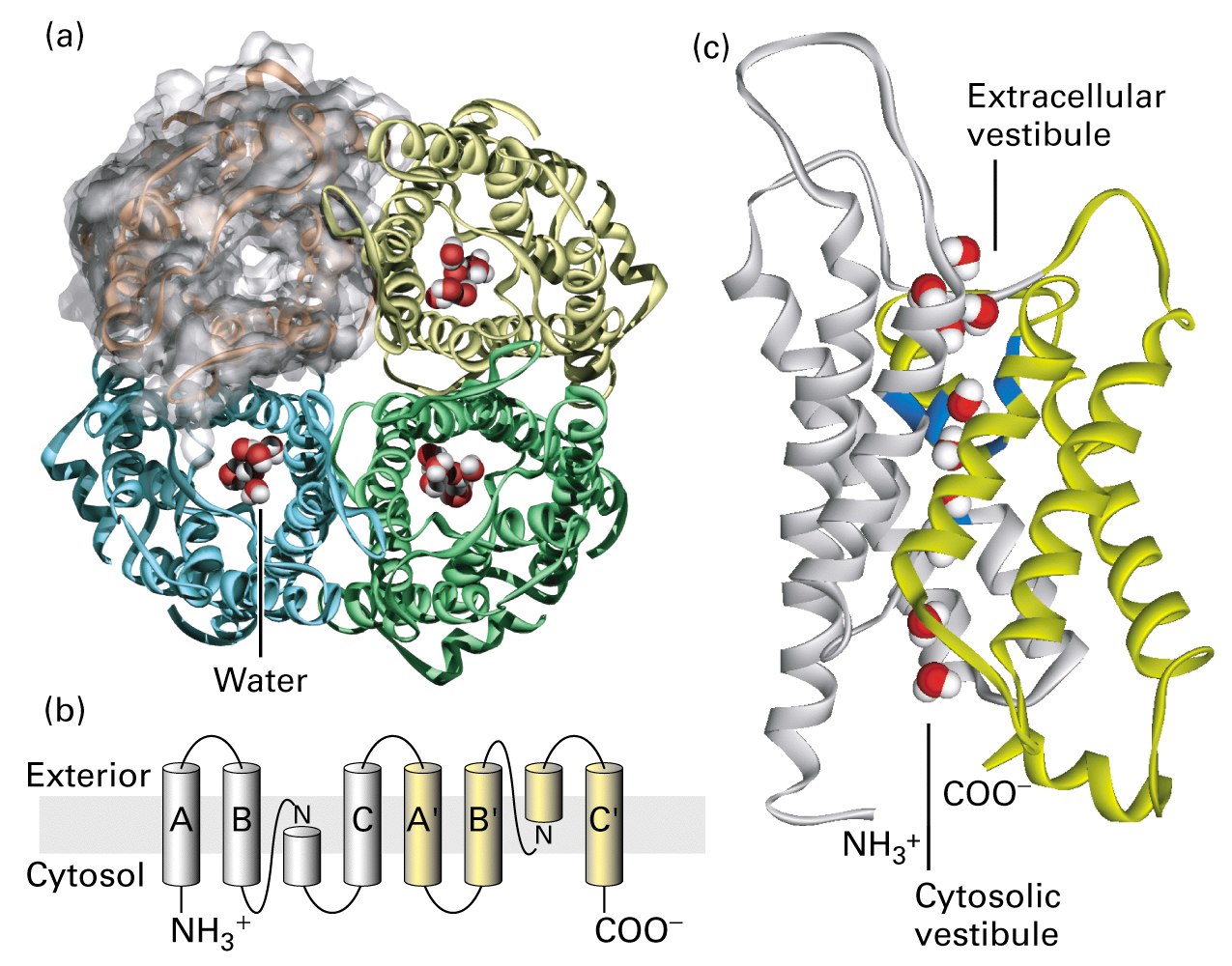
From Molecular Cell Biology
Aquaporin is one of the channels that allows water to cross the membrane.
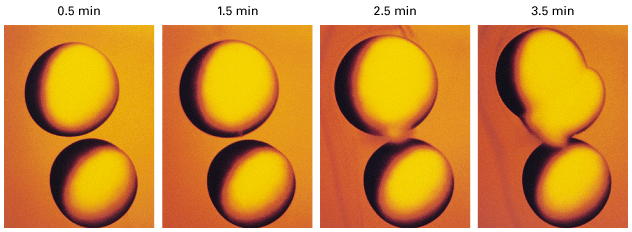
From Molecular Cell Biology
To test the function of aquaporin, mRNA from the cloned gene was injected into
Xenopus oocytes. Frog oocytes do not express aquaporin. These photographs show
control oocytes (bottom image in each panel) and microinjected oocytes (top
image in each panel) at the indicated times after transfer from an isotonic
salt solution (0.1 mM) to a hypotonic salt solution (0.035 mM).
The experimental oocytes swell because water now flows down its concentration
gradient into the oocytes.
From Molecular Biology of the Cell
Cystic fibrosis
Cystic fibrosis is a fatal disease caused by a genetic mutation a transport
protein. It is the most common lethal inherited disease of Caucasians, with
approximately one in 2500 newborns affected. This transport protein is found
in the lungs, pancreas, and sweat glands. The complication in the lungs is the
most severe problem in people with this disease.
The characteristic dysfunction in cystic fibrosis is the production of
abnormally thick sticky mucus by several types of epithelial cells. The
symptoms include respiratory disease due to the airways in the lungs being
blocked by thick plugs of mucus, usually followed by the development of
chronic bacterial infections.
The protein that is mutated in CF is called the cystic fibrosis transmembrane
conductance regulator (CFTR) and is a Cl- transporter. Cells isolated from CF
patients were shown to be defective in Cl- transport. Cells from normal lung
tissue secrete Cl-. The movement of Cl- from the cell causes Na+ to move into
the lung lumen and water passively follows these solutes. It is the movement
of water that is so necessary to keep the mucus lining the lungs hydrated and
thin. In the absence of Cl- transport, both Na+ and water transport doesn't
occur and the musus is abnormally thick.
CFTR was cloned
Back to home